- NEWS
- 07 December 2023
Landmark approval of the first CRISPR therapy paves the way for treatments based on more efficient and more precise genome editors.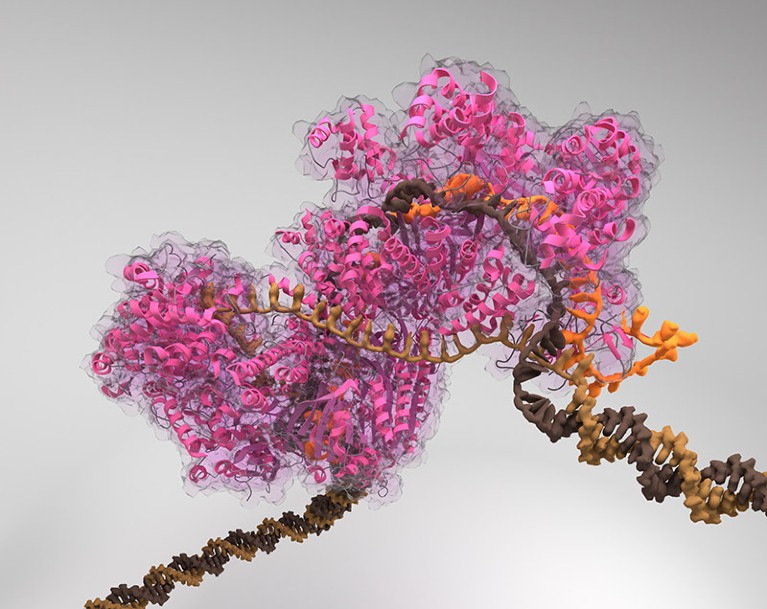
More versatile genome editors are supplanting the CRISPR-Cas9 editing system (artist’s illustration) for experimental treatments.Credit: Biolution GmbH/Science Photo Library
Less than a month after the world’s first approval of a CRISPR-Cas9 genome-editing therapy, researchers are hoping that the therapy will win its second authorization this week — this time from the United States, with its famously stringent regulators and lucrative health-care market.
The therapy, which UK regulators approved on 16 November, disables a gene as a means of treating a genetic blood disorder called sickle cell disease. A host of other CRISPR-Cas9 therapies that work on the same principle are in clinical trials as treatments for a range of diseases.
As sophisticated as these therapies are, they are only the beginning. “We tend to call these the first generation of genome editing,” says Keith Gottesdiener, chief executive officer of Prime Medicine, a company in Cambridge, Massachusetts, that is developing genome-editing therapies. “They can do some remarkable things, but they’re fairly limited.”
Now, however, there’s a fresh crop of CRISPR-based systems that overcome those limitations. These systems edit DNA with more precision and versatility than the original genome editors could achieve. And they can make changes, such as switching on genes, that the initial tools couldn’t. The regulatory approval of classical CRISPR-Cas9 “sets the stage” for the next generation of genome-editing techniques, says Marianne Carlon, a lung disease specialist at the Laboratory of Respiratory Diseases and Thoracic Surgery at KU Leuven in the Netherlands.
Here, Nature looks at the next generation of CRISPR techniques.
Base editing
Genome editing offers an opportunity to correct the mutations that cause cystic fibrosis, which affects the lungs and digestive system. But for that, classical CRISPR-Cas9 approaches are of little use: “CRISPR is much better at destroying things than it is at fixing things,” says Gottesdiener.Instead, Carlon is exploring a cystic-fibrosis treatment that harnesses a method called base editing, which can change individual DNA letters, or bases — converting an A to a G, for example, or a C to a T. Base editing relies on the Cas9 enzyme used in the original CRISPR system to target those changes to the correct spot. But unlike old-fashioned CRISPR-Cas9, base editing does not typically cut both strands of DNA at that spot. Instead, Cas-9 guides other enzymes to the chosen site, where they can go about the work necessary to change the DNA bases.
In the seven years since base editing was first reported, researchers have developed ways to reduce the number of unwanted DNA changes that it produces and shrink the size of its components so that they can be delivered more easily into cells. Base-editing therapies are already being used in early clinical trials, including atreatment for high cholesteroland a form of leukaemia. But the remarkable precision of the technique comes at the cost of inflexibility: it can be used to alter only certain DNA sequences, and cannot insert chunks of DNA into the genome.[/SIZE]
Prime editing
In 2019, a new CRISPR system called prime editing promised to address those limitations. Prime editing can change individual DNA bases, but can also either insert or delete small stretches of DNA at targeted sites. It is more flexible than base editing in that it can target and correct almost any site in the genome.But it is also more complicated. “There’s a lot of versatility, but that makes it a bit of a challenge to work with,” says Carlon.
Since 2019, researchers have made prime editing more efficient by designing better enzymes; other enhancements prevent the cell’s natural DNA-repair mechanisms from intervening and introducing errors.
Next year, Prime Medicine plans to seek permission from the US Food and Drug Administration to launch a clinical trial of a prime-editing treatment for chronic granulomatous disease, a genetic immune disorder.
Meanwhile, researchers are pushing the boundaries of the technique, devising ways to insert larger and larger pieces of DNA into targeted sites in the genome. This opens the door to replacing entire genes, says Omar Abudayyeh, a biological engineer at the Massachusetts Institute of Technology in Cambridge — thereby making it easier to develop a therapy to treat genetic disorders, such as cystic fibrosis, that can be caused by many different mutations within a certain gene. Instead of designing therapies to correct each mutation, it might one day be possible to replace the defective copy of the gene with a fresh one.
“Then you’d have a drug that’s applicable to every single patient for that disease,” he says. “Everybody’s working on different flavours of ways to do this.”
Epigenome editing
As well as altering the sequence of a gene itself, CRISPR systems can change how genes are expressed by altering the ‘epigenome’, including the array of chemical modifications to DNA that can affect gene activity.Technologies targeting the epigenome have not moved as quickly as base editing. In part, that’s because scientists assumed that epigenome edits would be erased during cell division, says Derek Jantz, chief scientific officer of Tune Therapeutics in Durham, North Carolina. “That’s a common misconception,” he says. “But epigenetics is very long-lasting.”
In May, scientists at Tune presented data showing that they could shut down a gene called PCSK9, which regulates cholesterol, in non-human primates without altering the bases in the DNA itself. Instead, they used a method that added chemical tags called methyl groups that are attached to the DNA and that regulate the activity of the gene. The effects have persisted for at least 11 months, says Jantz.
A long-lasting effect could give epigenome editing an advantage over some RNA-based medicines that must be readministered every few weeks or months. And the fact that the treatment doesn’t involve changing DNA relieves safety concerns that regulators have about CRISPR-Cas9 treatments, Jantz says.
The finding is also an example of how an improved understanding of the epigenome could push these treatments forwards, says Lei Stanley Qi, a synthetic biologist at Stanford University in California, and tackle diseases that other forms of CRISPR editing cannot. Tune, for example, hopes to use epigenome editing to treat hepatitis B virus infections, by silencing the viral DNA that can lurk in cells even after antiviral treatments.
Although such applications are a far cry from the CRISPR-Cas9 editing used in the first approved CRISPR medicine, the regulators’ approvals help to establish CRISPR-based editing as a viable way of treating disease, says Qi. That, in turn, could bolster interest in epigenome editing. “That approval is a huge deal,” he says. “After that, I guess we’ll enter a fast track.”
doi: https://doi.org/10.1038/d41586-023-03797-7